Coastal
Deltas, mangroves, & salt marshes
Deltas
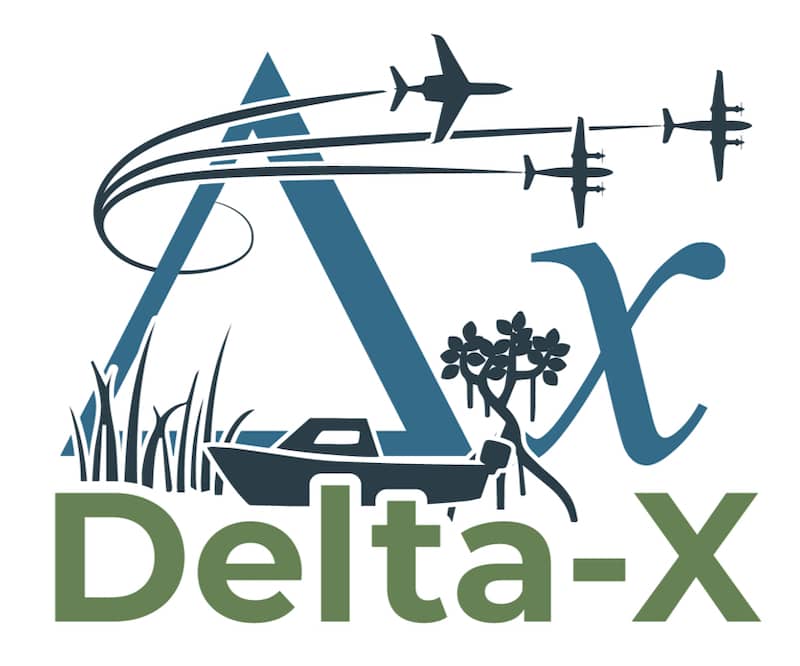
Dr. Simard is the principal investigator (PI) of the Delta-X mission (2019–2023). River deltas and their wetlands are drowning as a result of sea level rise and reduced sediment inputs. The Delta-X mission will determine which parts will survive and continue to grow, and which parts will be lost.
Delta-X’s objectives are to evaluate the roles of:
- Vegetation on soil accretion rates
- Channel-network density and deltaic island size on soil accretion rates
Methodology
- Investigate the Atchafalaya basin (part of the Mississippi Delta in the United States) using full physics hydrologic and ecogeomorphic numerical modeling, calibrated at the deltaic mesoscale with state-of- the-art remote-sensing and in situ observations;
- Model calibration is performed by calibrating model parameters to obtain water surface matching the remote sensing observations of water surface level, changes and slopes;
- Delta-X delivers fully calibrated hydrology and ecogeomorphic models of the basin.
Learn more at the Delta-X website.
Mangroves
Mangroves are forested wetlands that represent a functional link between the terrestrial and oceanic carbon cycles, storing up to four times as much carbon per unit area in comparison to terrestrial forest ecosystems.
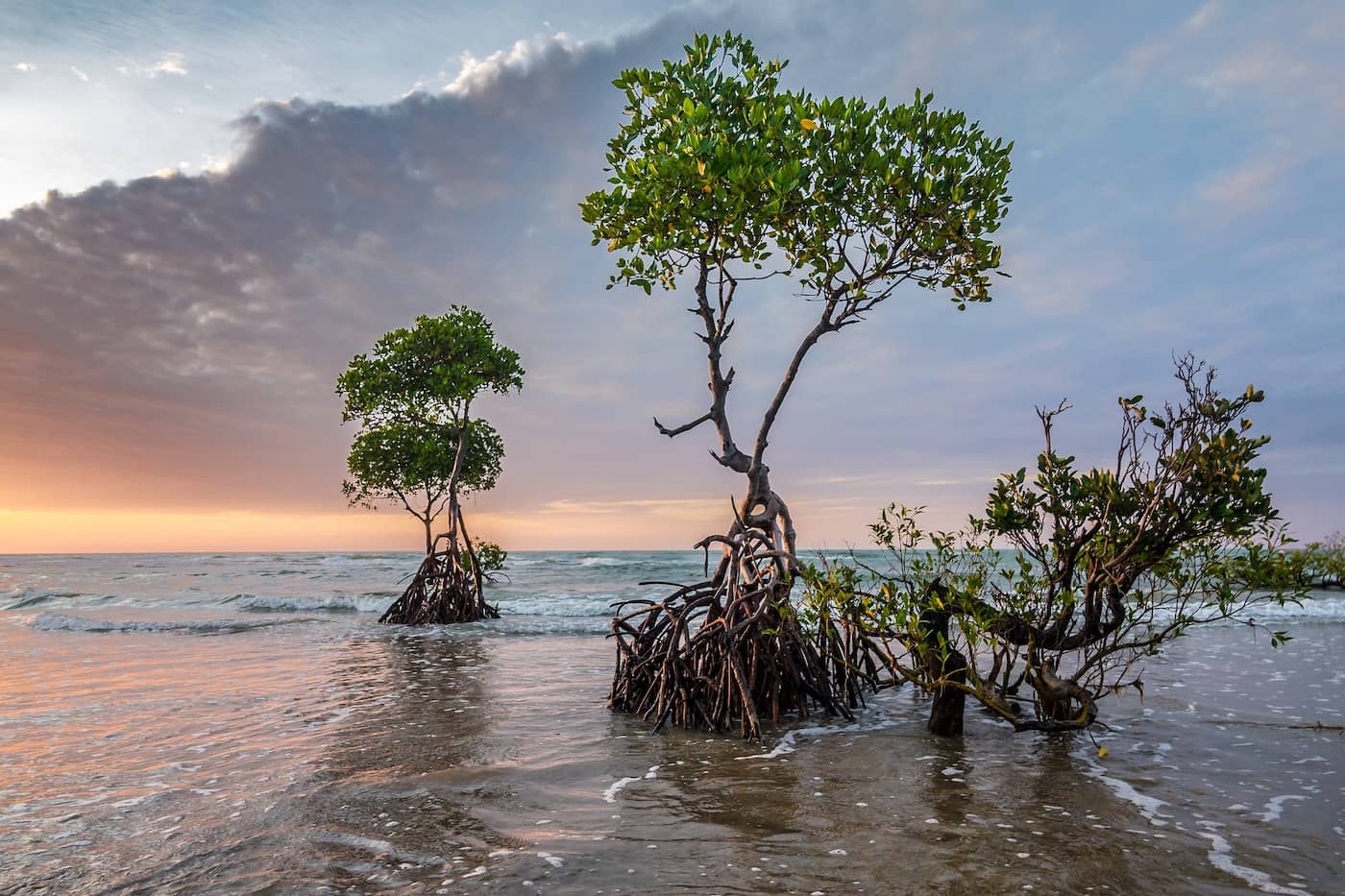
Mangroves contribute an estimated 10–15% of the global carbon storage in the coastal ocean, with ~50% of mangrove litterfall production being transported to adjacent coastal zones and accounting for 10–11% of the global export of particulate terrestrial carbon to the ocean.
Furthermore, mangrove forests provide a wealth of ecosystem services to coastal communities, including habitat for fisheries, firewood and timber, all valuable resources in local markets.
Despite this, mangroves are impacted by anthropogenically driven disturbances such as deforestation, conversion to aquaculture and urban development, and coastline transgression due to relative sea level rise.
Recent estimates of global mangrove loss rates range between 0.16% and 0.39% annually, and may be up to 8.08% in Southeast Asia. As a consequence, large amounts of previously stored carbon may be released into the atmosphere, contributing substantially to net global carbon emissions.
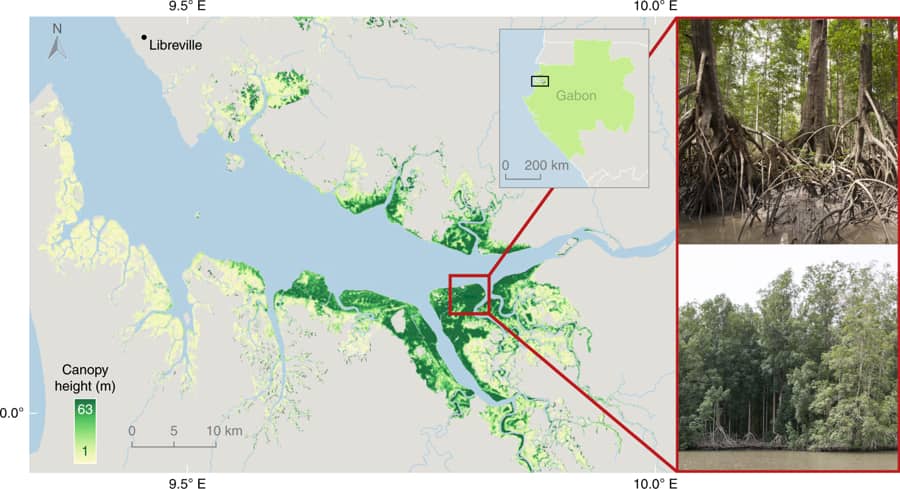
Mangrove forests in coastal Gabon are the tallest globally with forest stands that were estimated at 63 m. The photo insets show locations where individual trees were measured in situ up to 65 m tall.
We are mapping mangrove forest canopy height, biomass, and productivity and doing an assessment of vulnerability to anthropogenic activity and sea level change.
Salt marshes
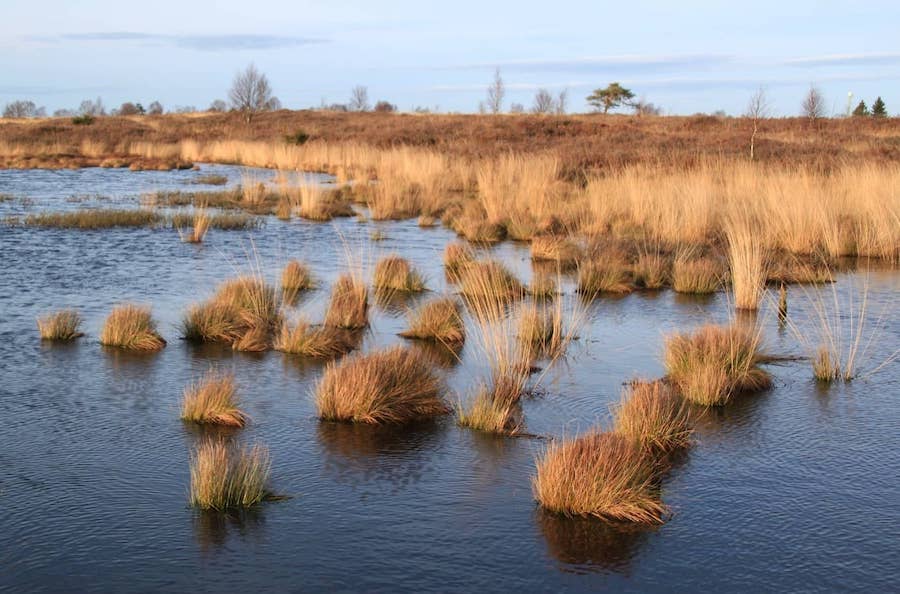
The soils and vegetation of coastal wetlands, including tidal marshes, mangroves and seagrasses, represent significant long-term standing carbon (C) pools that cumulatively sequester atmospheric carbon at annual rates comparable to terrestrial forest types despite their small global coverage. These C stocks and fluxes in intertidal environments are collectively referred to as “coastal wetland blue carbon.” In particular tidal marsh C stocks sequester carbon at rates of 1–2 Mg C per hectare per year on average, though are being converted to open water or other land cover types at rates of 1–2% globally. Conversion is primarily due to increasing coastal populations, agriculture and the effects of climate change, including sea-level rise and extreme weather events. Collectively, these contribute to greenhouse gas (GHG) emissions of 21–760 million Mg CO2eq per year.
Given their large C stocks and high carbon sequestration rates, as well as the potential for increased GHG emissions due to human conversion and degradation, coastal wetlands have in recent years received significant attention for their potential role in climate change mitigation. Entities interested in utilizing “coastal wetland blue carbon” as a management asset include voluntary C markets such as the Verified Carbon Standard (VCS). Approved VCS coastal wetland restoration and conservation projects can now receive carbon credits for reduction of GHG emissions.
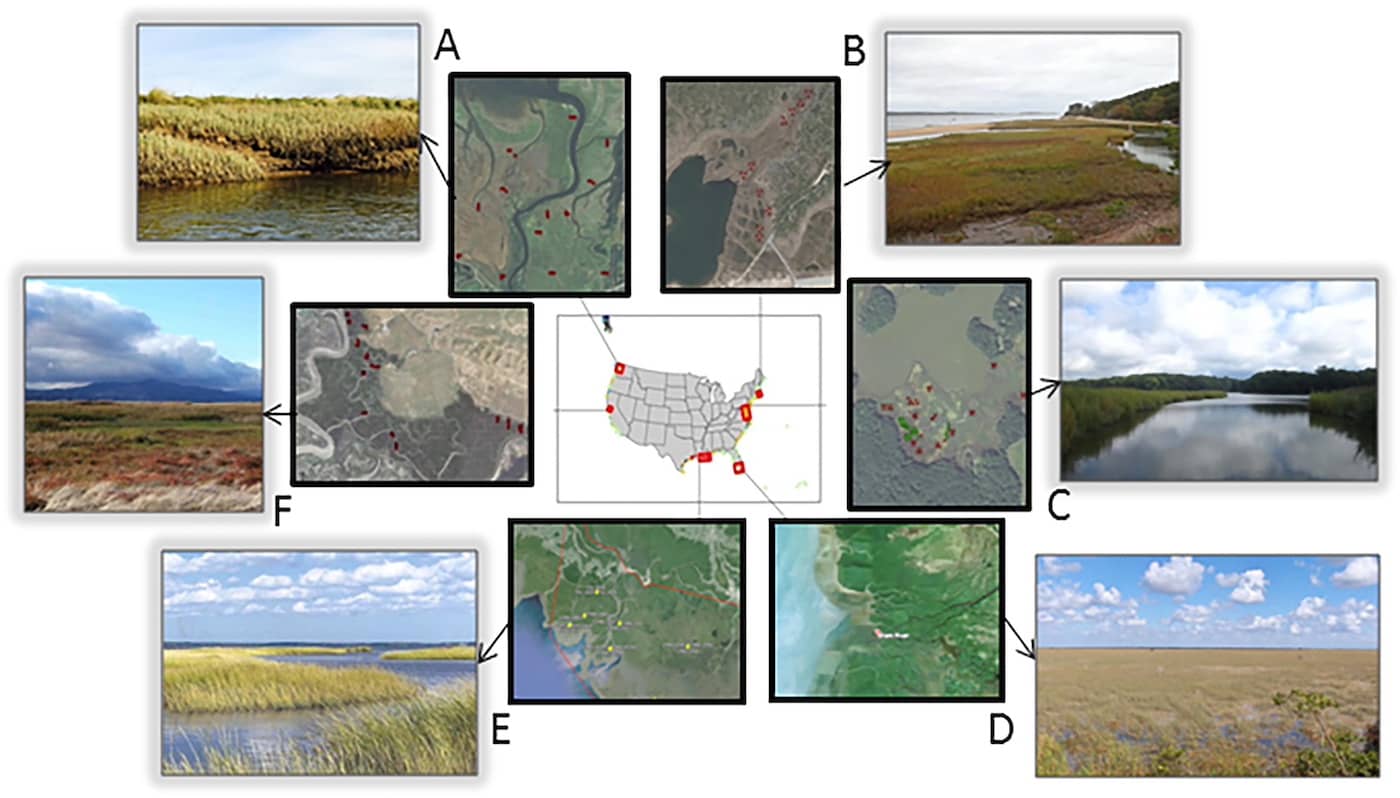
(A) Puget Sound, Washington (B) Cape Cod, Massachusetts (C) Chesapeake Bay, Maryland (D) Everglades, Florida (E) Mississippi River Delta, Louisiana (F) San Francisco Bay, California
Given differences in leaf morphology and plant growth form among emergent marsh species, our primary objective was to generate a single remote sensing model of tidal marsh aboveground biomass and carbon that represents nationally diverse saline, brackish and freshwater marshes. In order to successfully fulfill this, we developed the first national-scale dataset of aboveground tidal marsh biomass, species composition and aboveground plant C content from six U.S. estuaries representing a range of climate and salinity gradients. Using this dataset we test the performance of a repeatable remote sensing methodology for GHG accounting of tidal marsh aboveground C stocks that is consistent, transferable, free or low-cost and applicable to a range of estuarine characteristics.
Through the implementation of these objectives we address how the differences in plant community composition and vegetation structure across estuaries influence model development, and how additional or improved remote sensing data sets are able to improve model performance. In particular, we test the addition of Sentinel-1 C-band synthetic aperture radar backscatter with standard Landsat vegetation indices. We also explore how high resolution NAIP imagery can help to improve vegetation cover and biomass estimates. Given a final model, we evaluate uncertainties in mean aboveground C densities and total aboveground C across regions and wetland types mapped in C-CAP, and we investigate the capacity to discern regional and sub-regional differences in these estimates.
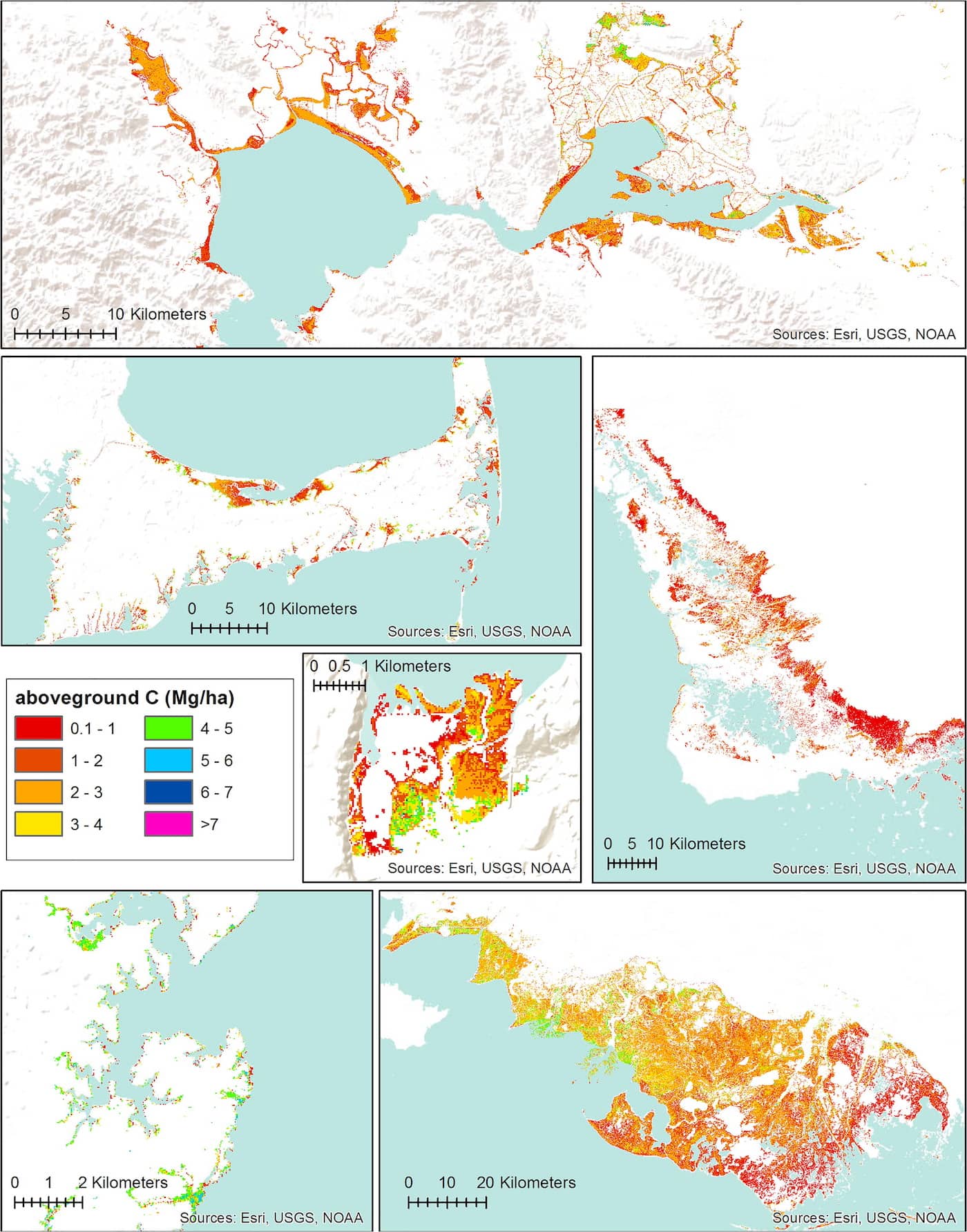
Tidal marsh aboveground carbon density maps of six study regions, based on the final biomass model (model #2, table 6) and plant percent carbon content data. Moving from top, left to right: San Francisco Bay, CA, Cape Cod, MA, Everglades, FL, Nisqually NWR, WA, Chesapeake Bay, MD, Terrebonne and St. Mary Parishes, LA.